Asking the right questions
Probably the most important skill of scientists is to develop a feeling for asking the right questions. This requires a thorough and deep understanding of their respective field and state-of-the-art knowledge. Asking the right questions as well implies to get a step beyond what is known and has been done, but based on a solid understanding and knowledge of what is readily available and what ought to be possible with minimal effort.Additionally, asking questions requires the scientists to have ample time to think about their data and projects. Hence, automating whatever is sensibly possible is the order of the day.
In this sense, the right question to ask is not “How can we make more people use EPR spectroscopy”. Developing EPR spectroscopy into a platform and tool for routine application is not an end in itself. Instead, think of the big questions of your research area and ask yourself: How could EPR spectroscopy help addressing these questions and obtain information (and possibly answers) other methods can not contribute?
Structure–function relationship of organic semiconductors
The applications listed below result from the authors’ own research and are mainly focussed on converting EPR spectroscopy into a routine analysis tool for organic semiconductors. Two fundamental aspects have been identified to determine the efficiency of organic semiconductors: morphology and electronic structure. However, these two rather fundamental characteristics can be translated into a list of different aspects of structure–function relationship of organic semiconductors, as shown in the figure below.
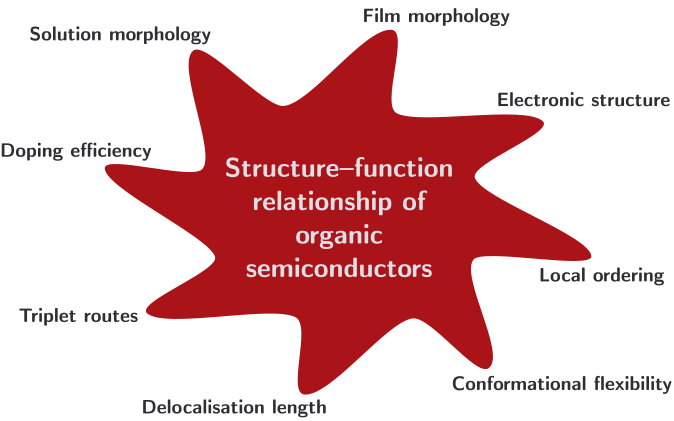
Aspects of the structure–function relationship of organic semiconductors. Each of these aspects can be and has been successfully investigated using EPR spectroscopy.
In the author’s lab, all these aspects have been investigated so far using “only” X-Band cw-EPR and TREPR spectroscopy at either room temperature or 80 K. The reason for not using pulsed EPR is quite simple: triplet states can only be measured with pulsed EPR methods at temperatures < 20 K. Therefore, the effort for cooling is much higher, and the insight gained usually not worth it. Similarly, investigating triplet states there is no need to go to higher fields, as their signals are entirely dominated by the zero-field splitting that can – for organic triplet states – usually be fully resolved at fields corresponding to X-band already.
Three large research areas can be identified:
doping of organic semiconductors
morphology in solution and film
electronic structure including delocalisation length and triplet routes.
For each of these, some more details of how EPR spectroscopy can contribute to answer outstanding questions are provided below, with further details provided separately.
Doping of organic semiconductors
Doping of organic semiconductors is of crucial importance particularly for organic field-effect transistors (OFETs) and thermoelectric generators, the first being already in widespread use and the second of increasing importance, particularly in light of the current climate crisis and the need for making much better use of energy sources available. While doping of organic semiconductors is widely applied, fundamental questions regarding the mechanisms involved are still to be answered.
Due to its inherent sensitivity to paramagnetic species and doped molecules usually possessing unpaired electron spins, EPR spectroscopy is a perfect match here.
Morphology in solution and film
Besides electronic structure, morphology of organic semiconductors has been identified as crucial for efficiency of organic electronic devices. Hence, gaining detailed insight into the morphology in thin films as well as in solution prior to casting is of outstanding interest. EPR spectroscopy provides unique capabilities due to its inherent molecular resolution and its ability to reveal preferential orientation of molecules in non-crystalline environments.
Questions that can be addressed using EPR spectroscopy include the effects of solvent, the overall order in noncrystalline films and the effect of side chains.
Electronic structure: delocalisation length and triplet routes
Electronic structure is the second crucial factor determining the efficiency of organic semiconductors, be it due to energy level matching of donor and acceptor materials in organic solar cells and organic light-emitting diodes or due to determining the transport properties for charges. Hence, a detailed understanding of the electronic structure on a molecular scale is of paramount importance.
Due to its molecular resolution, EPR spectroscopy provides unique insights into the electronic structure of organic semiconductors, including but not limited to revealing the role of donor and acceptor moieties in push–pull systems, the repeat unit in terms of electronic structure, factors determining exciton delocalisation length, and the origin of triplet states often encountered in these materials.
EPR method development
As can be seen from the above list of applications of EPR spectroscopy to address fundamental questions of organic semiconductors, there are two main types of EPR spectroscopy currently employed by the author:
cw-EPR spectroscopy (mostly at room temperature) for characterising doped organic semiconductors and investigating doping mechanisms, including preferential orientation of molecules and relative orientation of host and dopant
TREPR spectroscopy of triplet-states at 80 K for investigating the electronic structure and morphology of organic semiconductors by means of their light-induced triplet states.
While both have been quite successfully applied to the questions at hand, there is much room for improvement in order to make EPR spectroscopy a routinely employed analysis tool, mainly by automating both, data acquisition and analysis. Automating not only saves time, but greatly enhances robustness, reliability and accuracy of the results.
An incomplete list of (possible) applications from own experience and out of own interest.